Geothermal Heat Exchange
Welcome to the world of Geothermal Heat Exchange! This will guide you through the geothermal heating and cooling process, its applications, and its potential as an energy-efficient solution. Get ready to embark on your geothermal heat exchange learning journey!
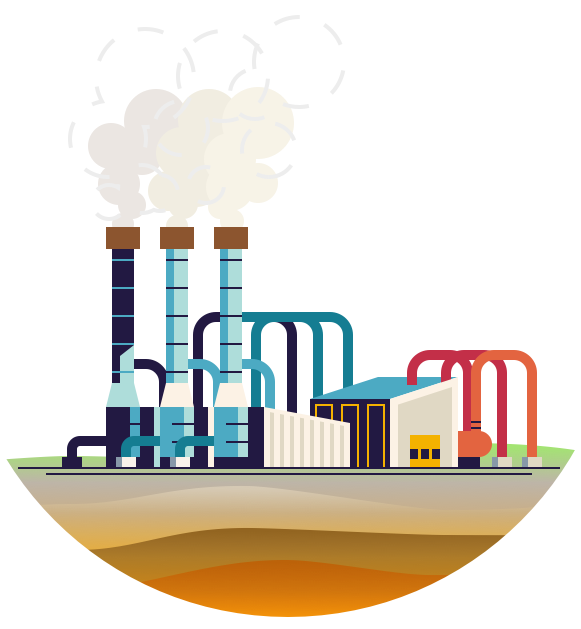
Topic Orientation
Introduction to Geothermal Heat Exchange
Geothermal heat exchange is a sustainable and energy-efficient method for heating and cooling buildings. It utilizes the stable temperature of the Earth to regulate indoor temperatures, reducing energy consumption and environmental impact.
Geothermal Heat Exchange Systems
Geothermal heat exchange systems consist of heat pumps and underground loops that transfer heat between the Earth and buildings. These systems offer an eco-friendly solution for heating, cooling, and hot water supply in residential and commercial structures, reducing utility costs and carbon emissions.
Geothermal Heat Exchange Benefits
Geothermal heat exchange offers numerous benefits, including reduced energy costs, lower greenhouse gas emissions, and independence from fossil fuels. It provides a reliable and sustainable solution for maintaining indoor comfort while contributing to environmental conservation.
Geothermal Heat Exchange Installation
Geothermal heat exchange systems require proper installation to function efficiently. Professional installers design and implement the underground loop system, connecting it to a heat pump within the building. The installation process includes drilling boreholes or trenches, ensuring proper loop spacing and depth for optimal heat transfer. Accurate installation is crucial to achieving the system's full potential in terms of energy efficiency and long-term reliability.
Geothermal Heat Exchange Maintenance
Maintenance tasks include inspecting the underground loops, checking for leaks, and ensuring the heat pump operates efficiently. Maintenance not only extends the system's lifespan but also helps maintain its energy-saving benefits. Routine inspections and servicing can prevent potential issues and reduce energy waste.
Environmental Impact and Sustainability
By relying on the Earth's natural heat, these systems significantly reduce carbon emissions compared to traditional heating and cooling methods. These systems use renewable energy and minimize the need for fossil fuels, contributing to a cleaner environment and a more sustainable future.
Application Tool
Step 1: Delta_T_logarithmic (ΔT Logarithmic)
The logarithmic temperature difference (ΔT Logarithmic) is a crucial parameter in geothermal heat exchange. It depends on the inlet temperature, outlet temperature, and ground temperature. Choose values that match your specific application.
Step 2: Heat Transfer Rate (q_dot)
The heat transfer rate (q_dot) is a key parameter in geothermal heat exchange. It depends on the mass flow rate of the fluid, specific heat capacity, and temperature variation. Select values that match your specific application.
Step 3: Length (length)
Calculate the length of the geothermal pipe based on the heat transfer rate, total thermal resistance, and ΔT Logarithmic.
Case Studies
Ball State University Geothermal System
Ball State University in Indiana, USA, implemented a geothermal system that heats and cools 47 buildings, covering 5.5 million square feet of space. This system saves approximately 45 million gallons of water, 500 billion British thermal Units (BTUs) of energy, and $2.2 – $2.5 million annually. The university has also sold carbon credits to fund sustainability and energy reduction efforts on campus.
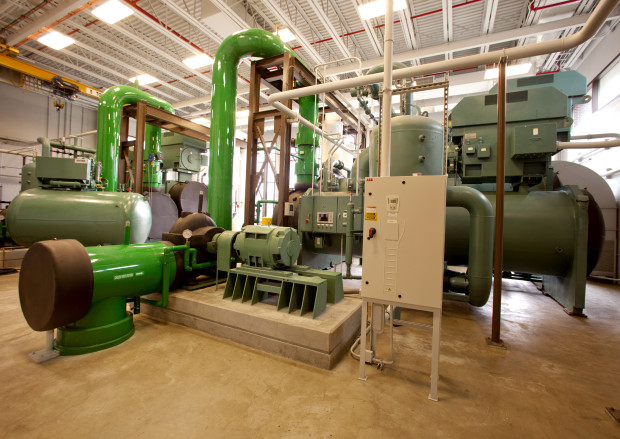
Reykjavik Geothermal District Heating
Reykjavik, the capital city of Iceland, has successfully implemented a district heating system using geothermal energy. The city utilizes the abundant geothermal resources in the region to provide heating and hot water to over 90% of its buildings. This system has significantly reduced the city’s reliance on fossil fuels and has contributed to a more sustainable and environmentally friendly heating solution.
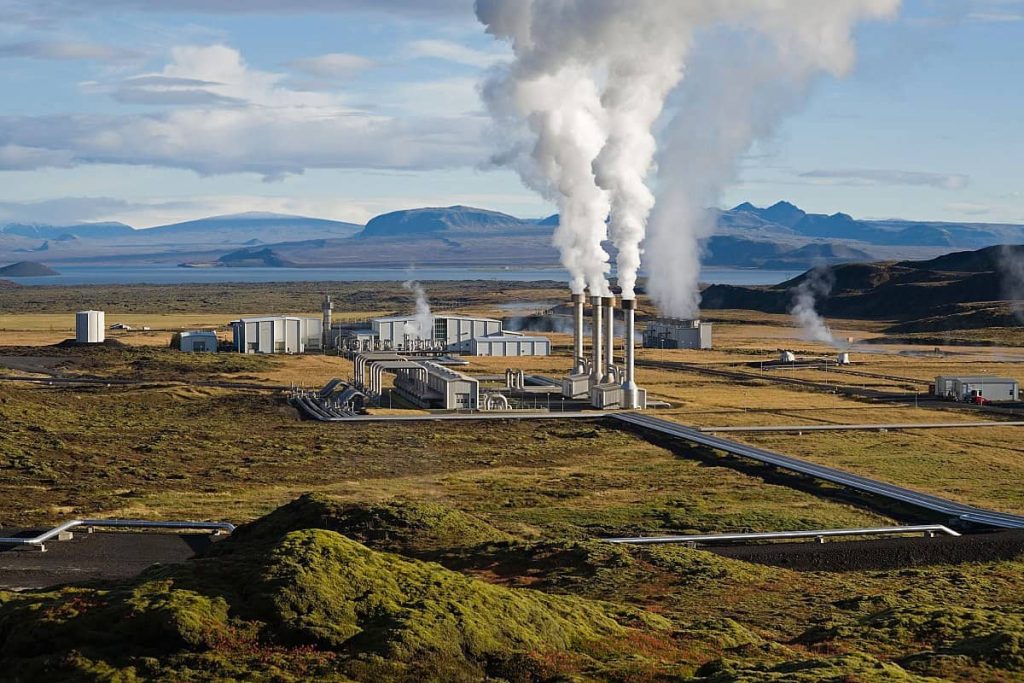
Hellisheidi Geothermal Power Plant
The Hellisheidi Geothermal Power Plant in Iceland is one of the largest geothermal power plants in the world. It harnesses the geothermal energy from the surrounding volcanic area to generate electricity and provide district heating to nearby communities. This plant has been instrumental in reducing Iceland’s dependence on fossil fuels and has become a model for geothermal power generation worldwide.
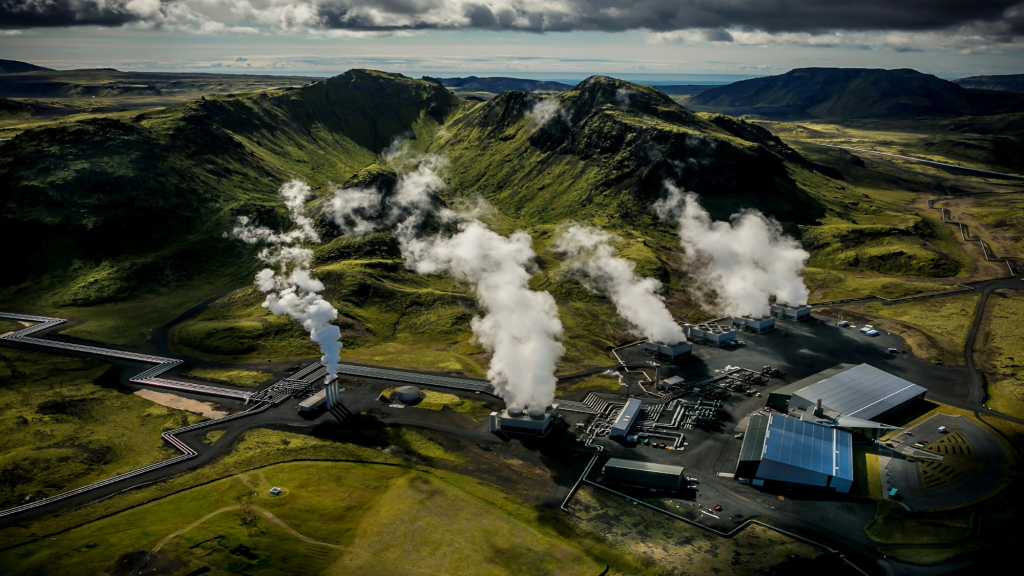
Visuals
Informative Visuals of Geothermal Heat Exchange.
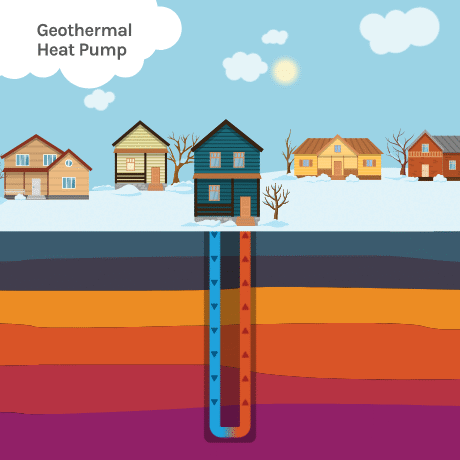

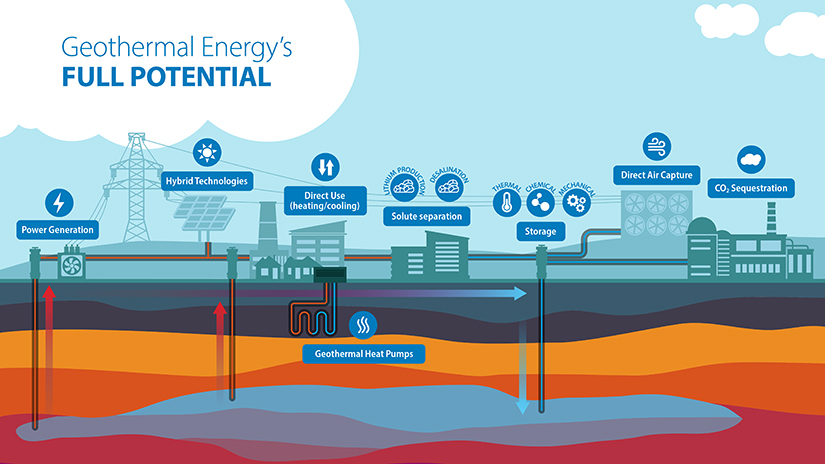
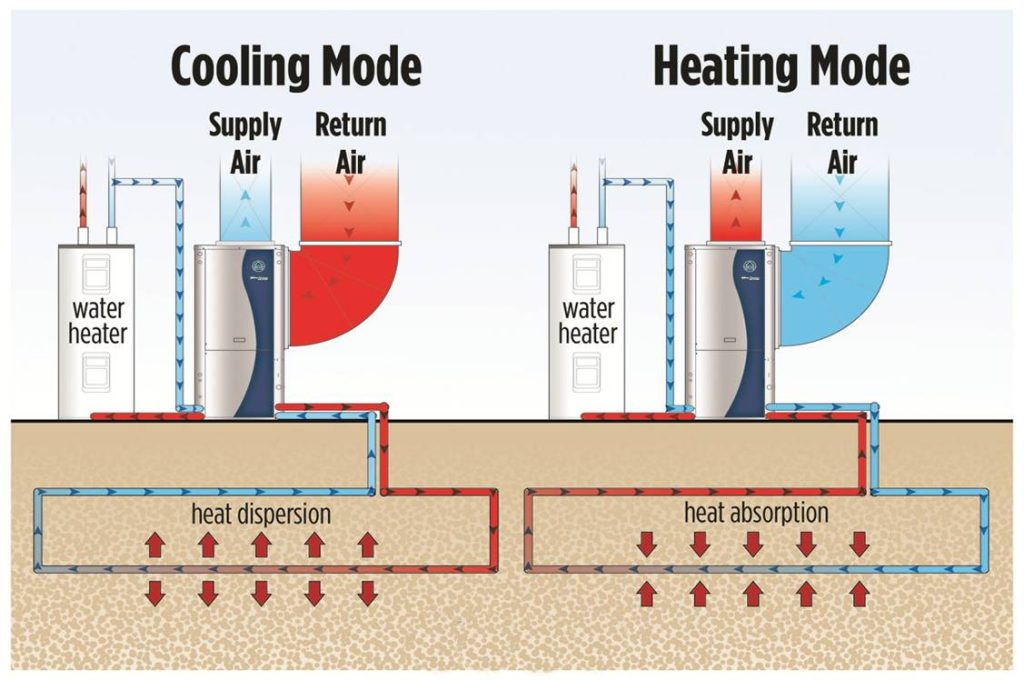
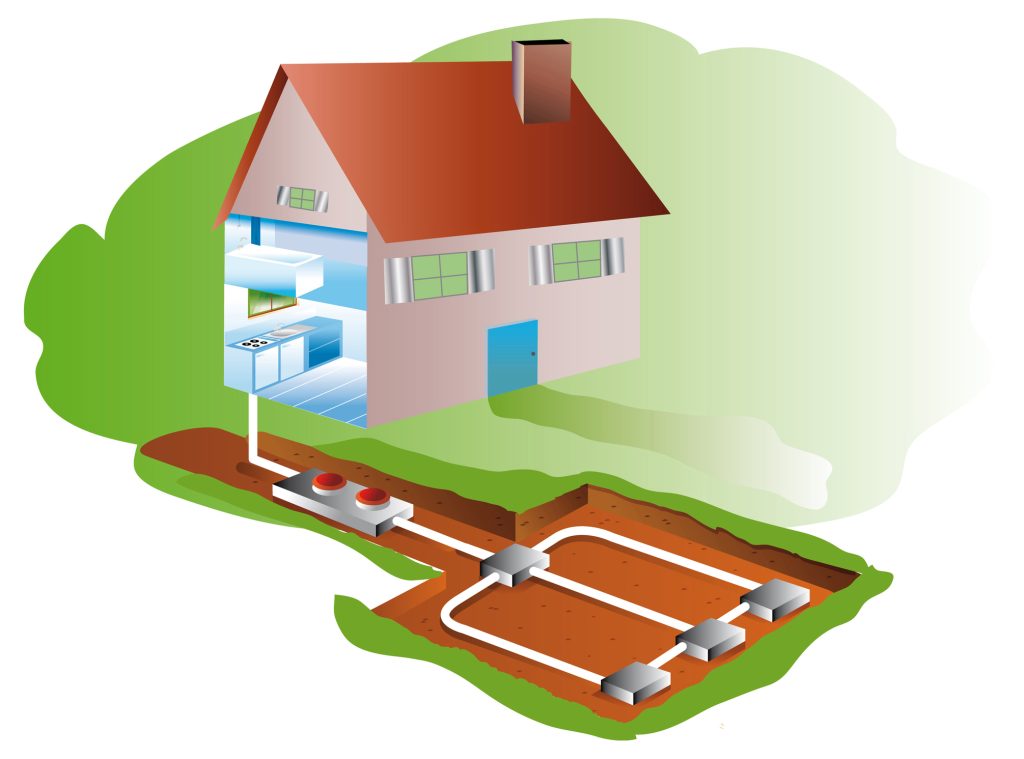
References
Here are some credible references discussing the subject of Geothermal Energy Systems.
Reference Title | Source |
---|---|
US Department of Energy – Geothermal Basics | Visit the US Department of Energy |
International Energy Agency – Geothermal Geoscience and Engineering for Sustainable Development | Explore the International Energy Agency’s Geothermal Resources |
Geothermal Energy Association – Wikipedia | Learn About the Geothermal Energy Association on Wikipedia |
National Renewable Energy Laboratory – Geothermal Energy Research | Access Geothermal Energy Research at NREL |
Sandia National Laboratories – Geothermal Research | Discover Geothermal Research at Sandia National Laboratories |
Stanford University – Geothermal Energy Research | Explore Stanford University’s Geothermal Energy Research |
Massachusetts Institute of Technology – Future of Geothermal Energy | Find Information on the Future of Geothermal Energy at MIT |
University of California, Berkeley – Geothermal Energy Systems Modeling | Learn About Geothermal Energy Systems Modeling at UC Berkeley |
International Geothermal Association – Love Geothermal | Visit the International Geothermal Association’s Website |
Geothermal Energy News – Latest Updates | Read the Latest Geothermal Energy News |
Equations
Here are some equations commonly used in the geothermal energy field:
- Heat Transfer Equation:
- Q = m * Cp * ΔT
This equation calculates the amount of heat transferred (Q) in a geothermal system, where m is the mass flow rate, Cp is the specific heat capacity, and ΔT is the temperature difference [1].
- Q = m * Cp * ΔT
- Power Generation Equation:
- Power = Q * Efficiency
This equation calculates the power generated by a geothermal power plant, where Q is the heat transfer and Efficiency is the overall efficiency of the power plant [1].
- Power = Q * Efficiency
- Carnot Efficiency Equation:
- Efficiency = 1 – (Tc/Th)
This equation calculates the maximum theoretical efficiency of a geothermal power plant based on the Carnot cycle, where Tc is the temperature of the cold reservoir and Th is the temperature of the hot reservoir [1].
- Efficiency = 1 – (Tc/Th)
- Reservoir Pressure Equation:
- P = P0 + ρ * g * h
This equation calculates the pressure (P) in a geothermal reservoir, where P0 is the initial pressure, ρ is the density of the fluid, g is the acceleration due to gravity, and h is the depth of the reservoir [1].
- P = P0 + ρ * g * h
- Fluid Flow Equation:
- Q = A * v
This equation calculates the fluid flow rate (Q) in a geothermal system, where A is the cross-sectional area and v is the velocity of the fluid [1].
- Q = A * v
- Geothermal Gradient Equation:
- ΔT/Δz = G
This equation represents the geothermal gradient (G), which is the change in temperature (ΔT) with respect to depth (Δz) in the Earth’s crust [1].
- ΔT/Δz = G
These equations are just a few examples of the mathematical relationships used in geothermal energy. They help engineers and researchers analyze and optimize geothermal systems for efficient and sustainable energy production.
Learn more:
Engineering Kit
Learn with practical experimentation using this educational hands-on engineering kit.
Quiz: Test Yourself
Your Reflection
Please feel free to document your inquiries, observations, queries, or any constructive feedback you may wish to provide.